Reactive power compensation systems work by dynamically adjusting the amount of reactive power in an electrical system to optimize performance, enhance power quality, and maintain voltage stability. The working principles vary depending on the type of technology used, but the core aim remains the same: managing reactive power to meet the needs of the power system efficiently. Here, I’ll detail how different types of reactive power compensation systems function:

Reactive Power
Compensation Systems
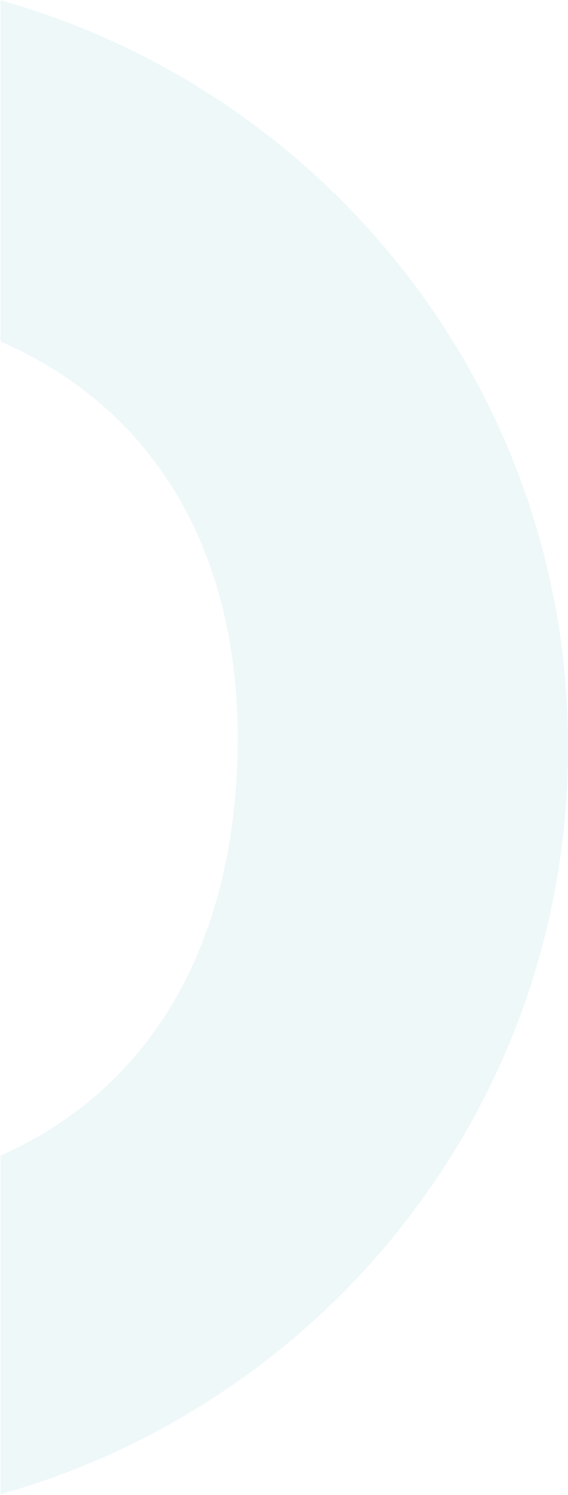
Capacitor Banks
Capacitor banks provide reactive power compensation by introducing capacitive reactive power into the system, which is especially useful for counteracting the inductive reactive power typically drawn by motors and transformers.
Functioning:
- Capacitors store electrical energy in the electric field created between their plates when a voltage is applied. In an AC system, capacitors have the effect of leading the current, which helps to neutralize the lagging current caused by inductive loads.
- By adding capacitors, the overall power factor of the system is improved towards unity, which means less reactive power is drawn from the supply. This reduction in reactive power demand leads to reduced losses in power transmission and distribution and improved voltage levels along the network.
Static Var Compensators (SVCs)
SVCs are fast-acting reactive power compensation devices that adjust the reactive power flow by switching in or out thyristor-controlled reactors and capacitor banks based on real-time system conditions.
Functioning:
- SVCs consist of thyristors, which are semiconductor devices used to switch electrical power circuits. By controlling the firing angle of the thyristors, SVCs can rapidly adjust the amount of capacitive or inductive reactive power being injected into or absorbed from the grid.
- This adjustment helps to stabilize voltage fluctuations caused by rapid changes in load and provides a more stable and reliable power supply.
Static Synchronous Compensators (STATCOMs)
STATCOMs use power electronics to provide a controllable source of reactive power based on voltage source converters (VSCs). They can respond faster and with greater control precision than SVCs.
Functioning:
- A STATCOM generates a voltage waveform through a VSC that is in phase and coupled with the grid via a reactor. By dynamically adjusting the amplitude and phase of this voltage relative to the system voltage, the STATCOM can either generate or absorb reactive power.
- This capability allows STATCOMs to support the voltage during both over-voltage and under-voltage conditions and manage dynamic and transient conditions in the grid more effectively.
Synchronous Condensers
Synchronous condensers are essentially synchronous motors or generators operated in a no-load condition but excited to provide or absorb reactive power.
Functioning:
- By adjusting the excitation of the synchronous condenser, its power factor can be shifted to either capacitive or inductive, allowing it to either supply reactive power to the grid or absorb excess reactive power.
- Synchronous condensers also contribute to the rotational inertia of the system, which is beneficial for overall grid stability, especially in power systems with a high penetration of non-inertial renewable energy sources.
Overall System Integration
- Control Systems: Modern reactive power compensation systems are integrated with sophisticated control systems that can detect voltage sags, swells, and other disturbances in real time. These systems calculate the required reactive power adjustment and command the compensation device accordingly.
- Monitoring and Maintenance: Ongoing monitoring ensures the compensation system is functioning effectively and helps in predicting maintenance needs, thereby reducing downtime and extending the service life of the equipment.
HARMONIC ANALYSIS
Harmonic analysis in a reactive power compensating system involves assessing the presence and characteristics of harmonic currents and voltages within the electrical network, particularly in relation to the operation of the reactive power compensation devices. Here’s how harmonic analysis is conducted in such systems:
Measurement of Harmonic Distortion:
- Harmonic analysis begins with the measurement of harmonic currents and voltages using specialized monitoring equipment such as power quality analyzers or harmonic meters.
- These instruments capture waveform data over a period of time, allowing engineers to identify harmonic components and assess their magnitudes, frequencies, and phase angles.
Identification of Harmonic Sources:
- The next step involves identifying the sources of harmonic distortion within the electrical system. This includes non-linear loads such as variable frequency drives (VFDs), rectifiers, arc furnaces, and other equipment that draw non-sinusoidal currents.
- By analyzing the harmonic spectra obtained from measurements, engineers can pinpoint the specific loads responsible for generating harmonics.
Assessment of Harmonic Impact:
- Harmonic analysis evaluates the impact of harmonic distortion on the performance and operation of the reactive power compensation system and the broader electrical network.
- High levels of harmonic distortion can lead to voltage distortion, increased losses, overheating of equipment, resonance problems, and interference with sensitive electronic devices.
Interaction with Compensation Devices:
- Harmonic analysis considers how reactive power compensation devices interact with harmonic currents and voltages in the system.
- Capacitor banks, SVCs, STATCOMs, and synchronous condensers may be susceptible to harmonic resonance or amplification if not properly designed or tuned to account for harmonic frequencies present in the system.
Mitigation Strategies:
- Based on the findings of harmonic analysis, mitigation strategies are developed to minimize the impact of harmonics on the performance of the reactive power compensation system and the overall power quality.
- This may involve filtering harmonic currents using passive harmonic filters, active harmonic filters, or hybrid filter solutions designed to target specific harmonic frequencies while maintaining effective reactive power compensation.
System Design and Optimization:
- Harmonic analysis informs the design and optimization of reactive power compensation systems to ensure they are robust, reliable, and compliant with relevant power quality standards and regulations.
- Proper sizing, placement, and configuration of compensation devices are critical to achieving effective harmonic mitigation without compromising system stability or reliability.
Continuous Monitoring and Maintenance:
- Ongoing monitoring of harmonic levels and system performance is essential to detect any changes or anomalies that may affect the effectiveness of the reactive power compensation system.
- Regular maintenance and periodic re-evaluation of the system design help to ensure optimal operation and mitigate potential risks associated with harmonics.
By conducting comprehensive harmonic analysis and implementing appropriate mitigation measures, reactive power compensating systems can effectively manage harmonic distortion, improve power quality, and enhance the overall performance and reliability of electrical networks.